Proximity effect shows potential for more efficient electronics
The newly discovered method of layering non-ferroelectric and ferroelectric materials could be applied to semiconductor and optoelectronic devices.
Monica Cooney
Jan 17, 2025
Ferroelectric proximity effect, when a non-ferroelectric material starts behaving like a ferroelectric material at the boundary where the two materials meet, has the potential to improve the components used in many technological applications. Materials that are ferroelectric have a spontaneous electric polarization that can be reversed by the application of an external electric field, and as such they are useful in data storage and wireless communication operations.
In a recent study published in Nature, Pennsylvania State University and Carnegie Mellon University researchers analyzed layered structures of non-ferroelectric materials (AlN and ZnO) and ferroelectric materials (Al1−xBxN, Al1−xScxN, and Zn1−xMgxO) in a variety of configurations. Using various testing methods, they confirmed that both layers could switch their electrical polarization, turning pure zinc oxide ferroelectric by stacking it with a ferroelectric material, such as magnesium-substituted zinc oxide thin films. The switching starts at the boundary in the ferroelectric layer and spreads to the non-ferroelectric layer, making it easier for the whole structure to change its polarization.
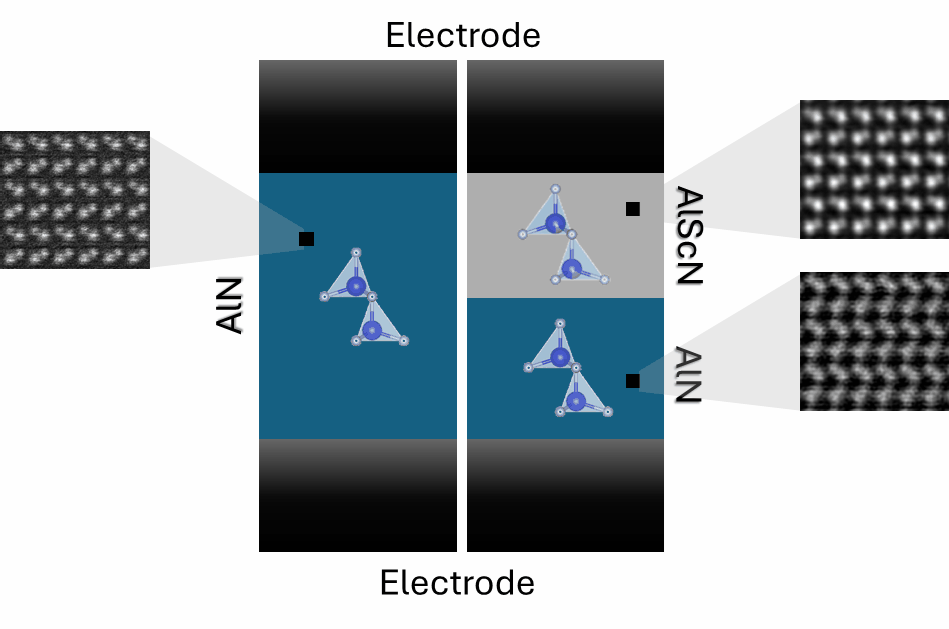
Source: Sebastian Calderon, CMU
Schematics illustrating electric-field-driven polarization reversal in AlN—a non-ferroelectric polar material (left panel)—induced by stacking it with one or more adjacent ferroelectric materials (right panel). The inset images show experimental differentiated differential phase contrast (dDPC) STEM images collected for a single-layer AlN (left panel) and for AlScN and AlN in an AlN/AlScN bilayer (right panel).
Contributors to the research from the CMU department of materials science and engineering included Department Head and Teddy and Wilton Hawkins Distinguished Professor Elizabeth Dickey, Professor Ismaila Dabo and Special Research Faculty Sebastian Calderon. This team also contributed to other recently reported research that provided insight into the switching mechanisms of AlN-based ferroelectrics.
As the amount of energy consumed by computing devices continues to grow and more efficient technologies are explored, this discovery is notable because it allows certain materials to become ferroelectric without changing their chemical makeup, which tends to degrade useful properties. The research also indicates that the switching layer can occupy as little as 3% of the total heterostructure volume, allowing the most-desirable material properties to represent the majority.
The findings open up new possibilities for integrating a wide variety of ferroelectric materials into next-generation semiconductor and optoelectronic devices.
Elizabeth Dickey, Department Head and Teddy and Wilton Hawkins Distinguished Professor, CMU Department of Materials Science and Engineering
“The findings open up new possibilities for integrating a wide variety of ferroelectric materials into next-generation semiconductor and optoelectronic devices,” said Dickey.
Understanding and controlling ferroelectric proximity effects is crucial for developing advanced nanoelectronic devices and exploring new avenues in materials science. One option to reduce energy consumption is changing the way processors talk to each other using light instead of electronics, noted Jon-Paul Maria, professor of materials science and engineering at Penn State and lead author of the study.
“This could mean that other enabling materials are already known, and exciting functional properties like ferroelectric switching just need unlocking using this proximity effect,” Maria said.
In addition to team members from Carnegie Mellon and Penn State, researchers from University of Pennsylvania and Oak Ridge National Laboratory also contributed to this research.
Carnegie Mellon University’s contribution to the research was supported by the Center for 3D Ferroelectric Microelectronics (3DFeM), an Energy Frontier Research Center funded by the US Department of Energy, Office of Science, Office of Basic Energy Sciences Energy Frontier Research Centers program under award DE-SC0021118.